Scientists at Johns Hopkins Medicine say they have developed an artificial lymph node with the potential to treat the cancer, according to a new study conducted on mice and human cells. The newly developed lymph node, a sac filled with immune system components, is implanted under the skin and is designed to act as a learning hub and stimulator to teach the immune system’s T cells to recognize and kill cancer cells.
Details of the experiments were recently published online at Advanced Materials.
The artificial lymph node that helps fight cancer
Lymph nodes, tiny glands scattered throughout the body, mainly in the neck, armpits and groin, are part of the immune system of mammals, including mice and humans. They number in the hundreds so that immune cells in one area of the body do not have to travel far to alert the immune system of imminent danger.
“They are a landing site where T cells, the cells that fight the immune system, lie dormant, waiting to be activated to fight infections or other abnormal cells,” says Natalie Livingston, Ph.D., first author of the research and currently researcher. postdoctoral fellow at Massachusetts General Hospital. “Because tumors can cause T cells to remain dormant, the artificial lymph node was designed to inform and activate T cells that are injected along with the lymph node.”
To create the artificial lymph node, scientists used hyaluronic acid, a moisturizing substance commonly used in cosmetics and lotions and found naturally in the skin and joints of the body.
Because of its properties, hyaluronic acid is often used in biodegradable materials such as wound healing patches intended to be implanted or applied to the body. Among these properties, hyaluronic acid can connect with T cells via a receptor on the cell surface.
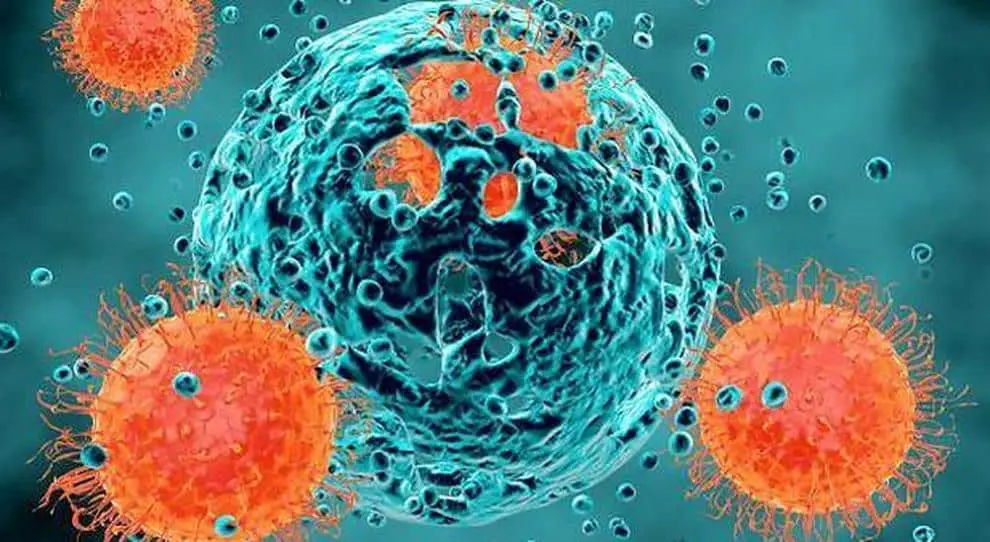
Johns Hopkins scientists led by Jonathan Schneck, M.D., Ph.D., published research in 2019 showing that hyaluronic acid increases T-cell activation.
For the current study, the Johns Hopkins team used hyaluronic acid as the scaffold, or base, for their new lymph node and added MHC (major histocompatibility complex) or HLA (human histocompatibility antigen) molecules, which stimulate T cells and other components of the immune system. Then, they also added molecules and antigens common to tumor cells to “teach” the T cells what to look for.
“By adding different antibodies to the artificial lymph node, we have the ability to control what the T cells are triggered to look for,” says Livingston.
The resulting artificial lymph node is about 150 microns in size, about twice the width of a human hair. It is small enough to stay under the skin and large enough to avoid being swept away into the bloodstream.
“An advantage of this approach over other cell therapies such as CAR-T is the fewer manufacturing steps,” says Schneck, director of Johns Hopkins University School of Medicine, professor of pathology, medicine and oncology. Center for Translational Immunoengineering and a member of the Institute for Cell Engineering, the Kimmel Cancer Center, and the Institute for Nanobiotechnology.
Current cell therapies require extracting T cells from a patient, manipulating them outside the body to recognize a particular type of cancer, and reinjecting them into the patient. “In our approach, we inject T cells together with an artificial lymph node, and the T cells are prepared and educated by the artificial lymph node inside the body. So, T cells can travel anywhere to destroy tumor cells,” says Schneck, who led the research team, along with Hai-Quan Mao, Ph.D., director of the Nanobiotechnology Institute at Johns Hopkins.
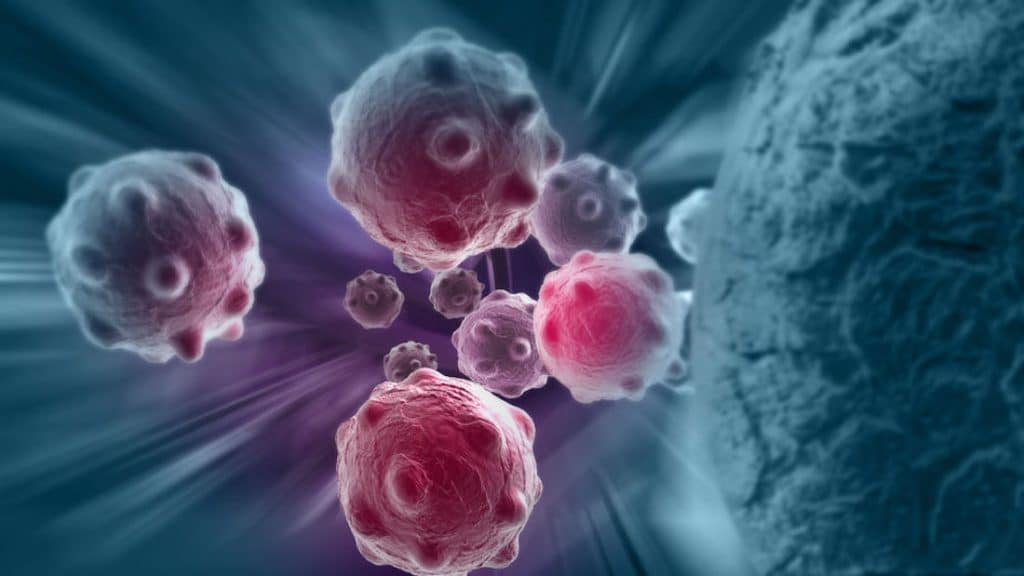
Livingston, Schneck and the research team tested the artificial lymph node in mice that had been implanted with melanomas or colon tumors. Six days after the tumors were implanted, the mice received injections of artificial lymph nodes and T cells.
The Johns Hopkins team compared these mice with those that received the artificial lymph node alone, those that received only T cells (which were not activated by the artificial lymph node), and those that received T cells in combination with a class of drugs immunotherapeutics called anti-PD-1.
Nine days later, mice with melanomas and colon tumors that received a combination of the artificial lymph node, T cells, and anti-PD-1 drug had the best survival rates (three of seven mice were still alive at 33 days), compared to other groups that only lived to about 26 days. This group of mice also had the slowest cancer growth rate. It took five to 10 days longer for their tumors to double in size compared to the other groups.
The scientists also found that the artificial lymph node attracts an influx of other immune cells and acts as an “immunologically active niche” to help further stimulate the immune system. When T cells were injected into mice next to the artificial lymph node, the number of T cells grew up to nine times more.
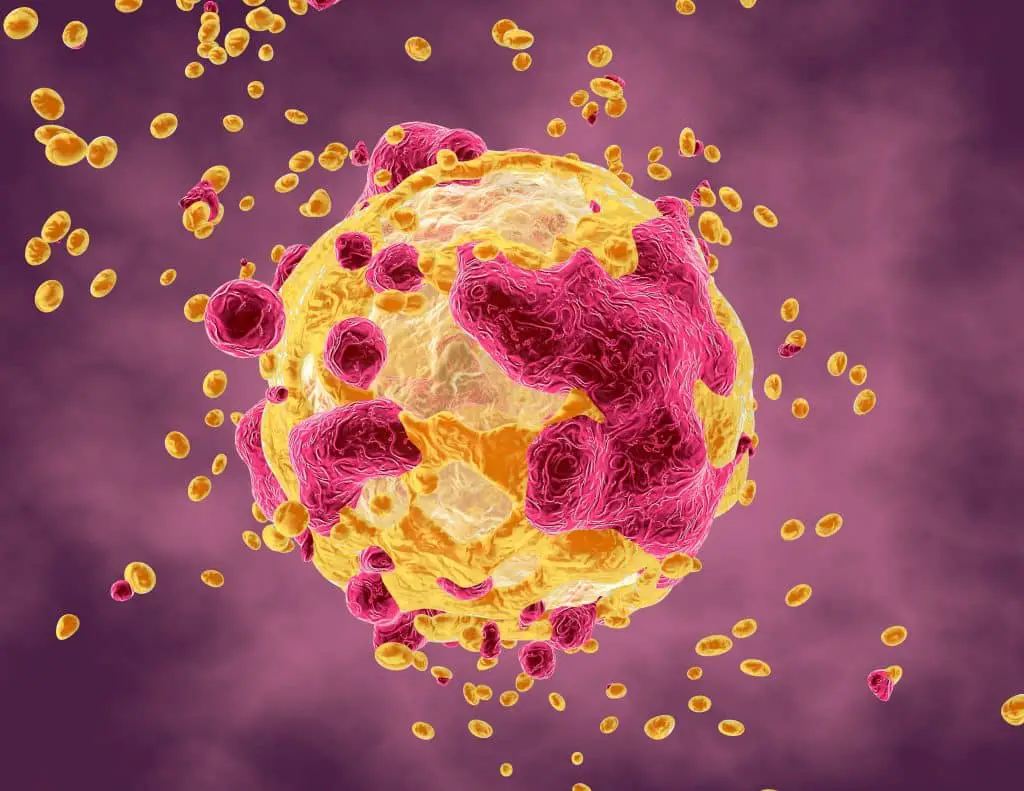
Livingston says the artificial lymph node approach is different from a cancer vaccine, which typically activates a dendritic cell, a component of the immune system that teaches T cells what to look for. People with cancer often develop malfunctioning dendritic cells, and the artificial lymph node bypasses the dendritic cell to directly activate the T cells.
The research team plans to conduct further laboratory studies to add more immune signaling molecules to the lymph node and recruit more host immune cells into the artificial lymph node environment.
“We have brought together the disciplines of materials science and immunology to create a potential therapy that forms its own immunological community, a kind of living drug,” says Schneck.
Scientists promote the creation of “artificial lymph nodes” to fight cancer and other diseases
In a proof-of-concept study in mice, Johns Hopkins Medicine scientists report the creation of a specialized gel that acts like a lymph node to successfully activate and multiply the immune system’s cancer-fighting T cells. The work brings scientists, they say, closer to injecting such artificial lymph nodes into people and activating T cells to fight disease.
In recent years, a wave of discoveries has advanced new techniques for using T cells, a type of white blood cell, in the treatment of cancer. To be successful, cells must be primed, or instructed, to detect and react to the molecular flags that dot the surfaces of tumor cells. The job of educating T cells in this way typically takes place in lymph nodes, small bean-shaped glands throughout the body that house T cells. But in patients with cancer and immune system disorders, the learning process is defective or does not happen.
To address such defects, current T-cell enhancement therapy requires doctors to remove T cells from a cancer patient’s blood and reinject the cells into the patient after genetically engineering or activating the cells in a laboratory so that they recognize cancer. connected molecular flags.
One such treatment, called CAR-T therapy, is expensive and available only at specialized centers with laboratories capable of the complicated task of engineering T cells. Furthermore, it typically takes six to eight weeks to culture T cells in laboratories and, Once reintroduced into the body, the cells do not last long in the patient’s body, so the effects of treatment may be short-lived.
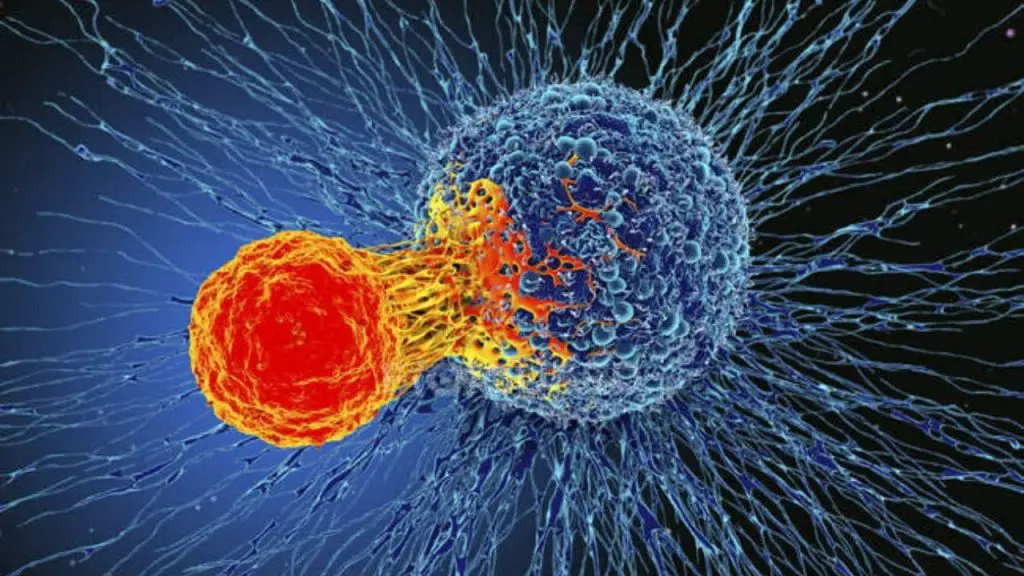
The new work, reported April 10 in the journal Advanced Materials, is an attempt by Johns Hopkins scientists to find a more efficient way to engineer T cells.
“We believe that the T cell environment is very important. Biology doesn’t show up on plastic plates; it happens in tissues,” says John Hickey, Ph.D. candidate in biomedical engineering at the Johns Hopkins University School of Medicine and first author of the study report.
To make the engineered T cell environment more biologically realistic, Hickey, working with his mentors Hai-Quan Mao, Ph.D., associate director of the Johns Hopkins Institute for NanoBioTechnology, and Jonathan Schneck, M.D., Ph.D., professor of pathology, medicine and oncology at the Johns Hopkins University School of Medicine, tried to use a gelatin polymer, or hydrogel, as a platform for T cells. On the hydrogel, the scientists added two types of signals that stimulate and “teach ” to T cells to focus on foreign targets to destroy.
In their experiments, T cells activated on hydrogels produced 50% more molecules called cytokines, a marker of activation, than T cells kept on plastic culture dishes.
Because hydrogels can be made to order, Johns Hopkins scientists have created and tested a range of hydrogels, from the very soft feel of a single cell to the stiffer quality of a cell-rich lymph node.
“One of the surprising findings was that T cells prefer a very soft environment, similar to interactions with single cells, compared to densely packed tissue,” Schneck says.
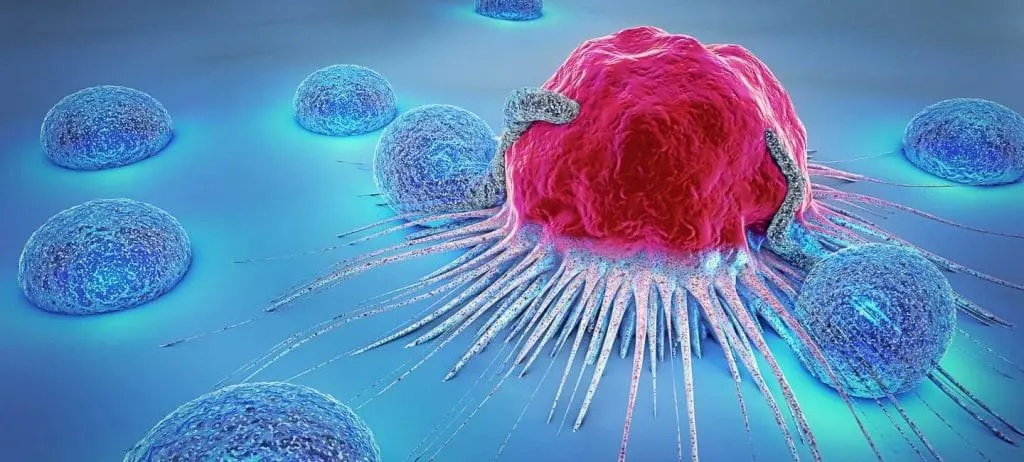
More than 80% of the T cells on the soft surface multiplied, compared to none of the T cells on the firmer type of hydrogel.
When the Johns Hopkins team placed T cells on a soft hydrogel, they found that the T cells multiplied from a few cells to about 150,000 cells, enough for cancer therapy, within seven days. In contrast, when scientists used other conventional methods to stimulate and expand T cells, they were only able to grow 20,000 cells within seven days.
In the next series of experiments, the scientists injected the engineered T cells into soft hydrogels or plastic culture dishes into mice with melanoma, a lethal form of skin cancer. Tumors in mice with T cells grown on hydrogels remained stable in size, and some mice survived beyond 40 days. In contrast, tumors grew in most of the mice injected with T cells grown in plastic dishes, and none of these mice lived beyond 30 days.
As we refine the hydrogel and replicate essential features of the natural environment, including chemical growth factors that attract cancer-fighting T cells and other signals, we will eventually be able to design artificial lymph nodes for cell-based regenerative therapy. immunology,” says Schneck, a member of the Johns Hopkins Kimmel Cancer Center.
The scientists have filed patents related to the hydrogel technology described in their report.
#Artificial #lymph #node #developed #potential #cure #cancer