Gravitational waves, ripples in space-time predicted by Einstein nearly a century ago, were first detected in 2015. A new study led by Yanou Cui, associate professor of physics and astronomy at the University of California, Riverside, reports that very simple forms of matter could create detectable gravitational-wave backgrounds soon after the Big Bang.
Gravitational waves detectable
This mechanism of creating detectable gravitational wave backgrounds could shed light on the mysterious gravitational wave signal recently captured by pulsar timing observatories,” Cui said. “Another interesting implication is that the same form of matter could be identified as dark matterthe mysterious substance thought to make up most of the mass in the universe and which has puzzled scientists for decades.”
The study, published in Physical Review Letterspaves the way for the discovery of new fundamental physics using the greatest laboratory: the Universe itself.
The simplest form of matter is a type of ultralight scalar field matter. Scalar means that the matter has no internal rotation (spin) and resembles the Higgs boson. These forms of matter are very light, each with a mass of a millionth or even a billionth of the mass of an electron. Because of their very small mass, they behave more like waves than particles and permeate the Universe.
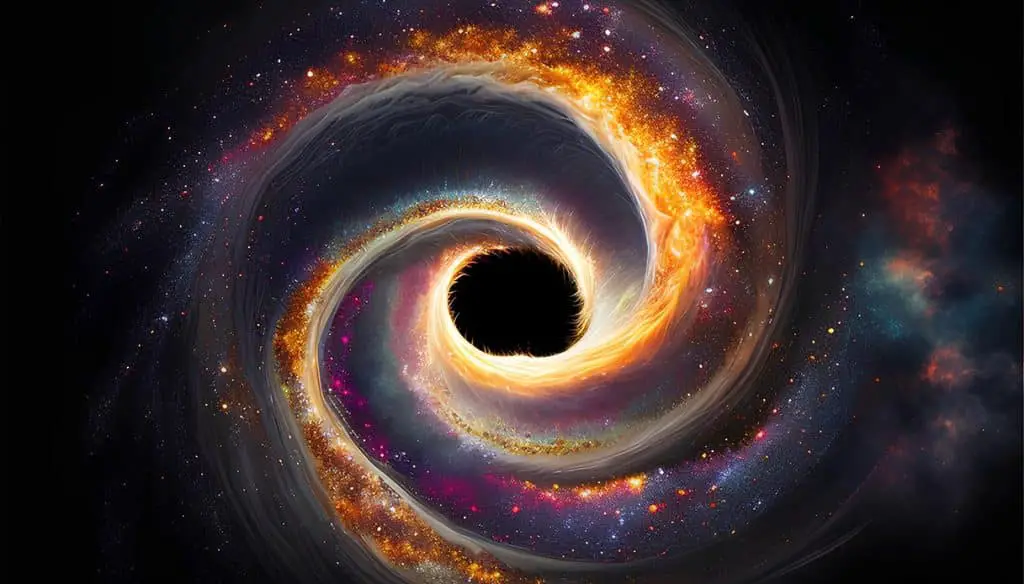
Gravitational waves (GWs) must be of sufficiently high intensity, analogous to electromagnetic waves, for current experiments to be sensitive enough to capture them. Furthermore, they must be in the frequency bands to which these experiments are sensitive; so far, only certain frequency ranges of GWs can be detected due to technological limitations.
GWs are generated during an era when the expansion rate of the universe is around the mass of the scalar field. It would stop beyond this point because the particle production would be stopped at some point by the internal machinery. When I say shortly after the Big Bang, it is still a fraction of a second later. Long after the Big Bang, there could be GW production from astrophysical sources such as black hole mergers, which the Laser Interferometer Gravitational-wave Observatory has observed.
They don’t interact with known matter in any way other than super-weak gravitational interaction. The GW signal we demonstrated is the way to detect them. But if they are dark matter, we knew about them from the dark matter general evidence. Again, since they only interact with visible matter gravitationally, which is very weak, we didn’t know much about them before.
This is one of the highlights. But it is also highly non-trivial to produce detectable GWs, and we have found that a simple form of matter, perhaps dark matter, can produce such GWs, a significant theoretical result. Furthermore, as mentioned earlier, last year’s puzzling discovery of pulsar timing can be explained by this mechanism.
Gravitational waves could reveal the nature of dark matter
Observations of gravitational waves from merging black holes could reveal new insights into dark matter, suggests a new study by an international team led by UCL.
The study, presented at the National Astronomy Meeting in Cardiff and now published in the journal Physical Review D, used computer simulations to study the production of gravitational-wave signals in simulated universes with different types of dark matter.
Their findings show that counting the number of black hole mergers detected by the next generation of observatories could tell us whether or not dark matter interacts with other particles and thus help us understand what it is made of.
Cosmologists generally regard dark matter as one of the biggest missing pieces in our understanding of the cosmos. Despite strong evidence that dark matter makes up 85% of all matter in the universe, there is currently no consensus on its underlying nature. This includes questions such as whether dark matter particles can collide with other particles such as atoms or neutrinos, or whether they pass through them unaffected.
One way to test this is to observe how galaxies form in dense clouds of dark matter called halos. If dark matter collides with neutrinos, the structure of the dark matter is broken down, resulting in fewer galaxies forming.
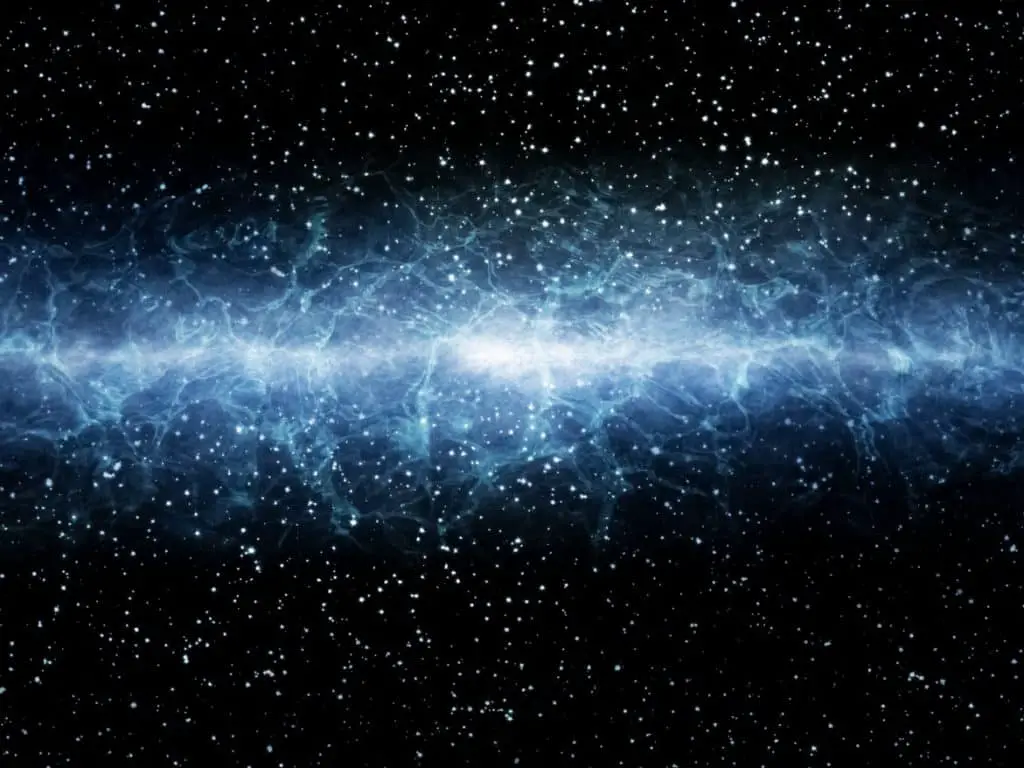
The problem with this method is that the disappearing galaxies are very small and very distant from us, so it is difficult to see whether they are there or not, even with the best telescopes available.
Instead of targeting the missing galaxies directly, the authors of this study propose using gravitational waves as an indirect measure of their abundance. Their simulations show that in models where dark matter collides with other particles, there are significantly fewer black hole mergers in the distant universe.
Although this effect is too small to be observed by current gravitational-wave experiments, it will be a primary target for the next generation of observatories currently being designed.
The authors hope that their methods will help spark new ideas about using gravitational-wave data to explore the large-scale structure of the universe and shed new light on the mysterious nature of dark matter.
Dr Alex Jenkins (UCL Physics & Astronomy), one of the study’s lead authors, said: “Gravitational waves are a powerful new tool for observing the distant Universe. The next generation of observatories will detect hundreds of thousands of black hole mergers every year, giving us unprecedented insights into the structure and evolution of the cosmos.”
Co-author Dr Sownak Bose from Durham University said: “Dark matter remains one of the enduring mysteries in our understanding of the Universe. This means it is particularly important that we continue to identify new ways to explore models of dark matter, combining both existing and new probes to best test the model’s predictions. Gravitational-wave astronomy offers a path to better understand not only dark matter, but also the formation and evolution of galaxies in general.”
Using Gravitational Waves to Hunt for Dark Matter
Using computer simulations, an international team of cosmologists has found that observations of gravitational waves from merging black holes could reveal the true nature of dark matter. Their discovery was presented at the National Astronomy Meeting by co-author Dr. Alex Jenkins of University College London.
The team used computer simulations to study the production of gravitational-wave signals in simulated universes with different types of dark matter. Their findings show that counting the number of black hole mergers detected by the next generation of observatories could tell us whether or not dark matter interacts with other particles, giving us new insights into what it’s made of.
Cosmologists generally regard dark matter as one of the biggest missing pieces in our understanding of the cosmos. Despite strong evidence that dark matter makes up 85% of all matter in the universe, there is currently no consensus on its underlying nature. This includes questions such as whether dark matter particles can collide with other particles such as atoms or neutrinos, or whether they pass through them unaffected.
One way to test this is to observe how galaxies form in dense clouds of dark matter called halos. If dark matter collides with neutrinos, the structure of the dark matter is broken up, resulting in fewer galaxies forming. The problem with this method is that the galaxies that disappear are very small and very distant from us, so it is difficult to see whether they are there or not, even with the best telescopes available.
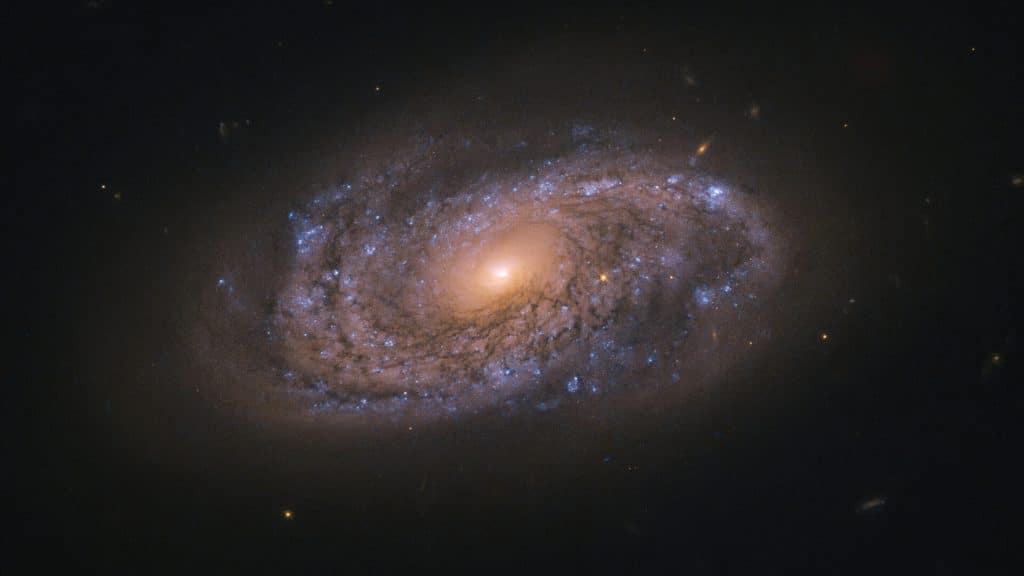
Instead of targeting the missing galaxies directly, the authors of this study propose using gravitational waves as an indirect measure of their abundance. Their simulations show that in models where dark matter collides with other particles, there are significantly fewer black hole mergers in the distant universe. While this effect is too small to be seen by current gravitational-wave experiments, it will be a prime target for the next generation of observatories currently being designed.
The authors hope that their methods will help spark new ideas about using gravitational-wave data to explore the large-scale structure of the universe and shed new light on the mysterious nature of dark matter.
Co-author Dr Sownak Bose from Durham University said: “Dark matter remains one of the enduring mysteries in our understanding of the Universe. This means it is particularly important that we continue to identify new ways to explore dark matter models, combining both existing and new probes to best test the model’s predictions. Gravitational-wave astronomy offers a path to better understand not only dark matter, but also the formation and evolution of galaxies in general.”
Markus Mosbech of the University of Sydney, another co-author, adds: “Gravitational waves offer us a unique opportunity to observe the early Universe, as they pass through the Universe unobstructed, and next-generation interferometers will be sensitive enough to detect single events at large distances.”
Another member of the research team, Professor Mairi Sakellariadou from King’s College London, said: “The third-generation gravitational wave data will provide a new and independent way to test the current model of the evolution of our universe and shed light on the still unknown nature of dark matter.”
#Gravitational #Waves #Explain #Dark #Matter #Mysteries #Big #Bang