The star-shaped glial cells, the so-called astrocytesare more than just a support cell in the brain. They are actively involved in learning processes and interact with nerve cells. But what exactly do they do?
The function of astrocytes
Researchers at the University Hospital Bonn (UKB) and the University of Bonn are using a biophysical model to clarify how glial cells interact with nerve cells to regulate rapid adaptation to new information. The results of the study have now been published in Communications Biology .
In the brain, synaptic plasticity, or the ability to change neuronal connections over time, is critical to learning and memory. Traditionally, science has focused on nerve cells and their synapses. The discovery of intracellular Ca 2+ signaling in astrocytes has led to the idea that astrocytes are more than the glue that holds the brain together and play a crucial role in this process.
“Dysfunction of astrocytes can significantly impair our ability to learn, highlighting their importance in cognitive processes. However, the exact functions of astrocytes have long remained a mystery,” says corresponding and co-senior author Prof. Tatjana Tchumatchenko, research group leader at the Institute for Experimental Epileptology and Cognitive Research at the UKB and member of the Transdisciplinary Research Area (TRA) “Modeling” at the University of Bonn.
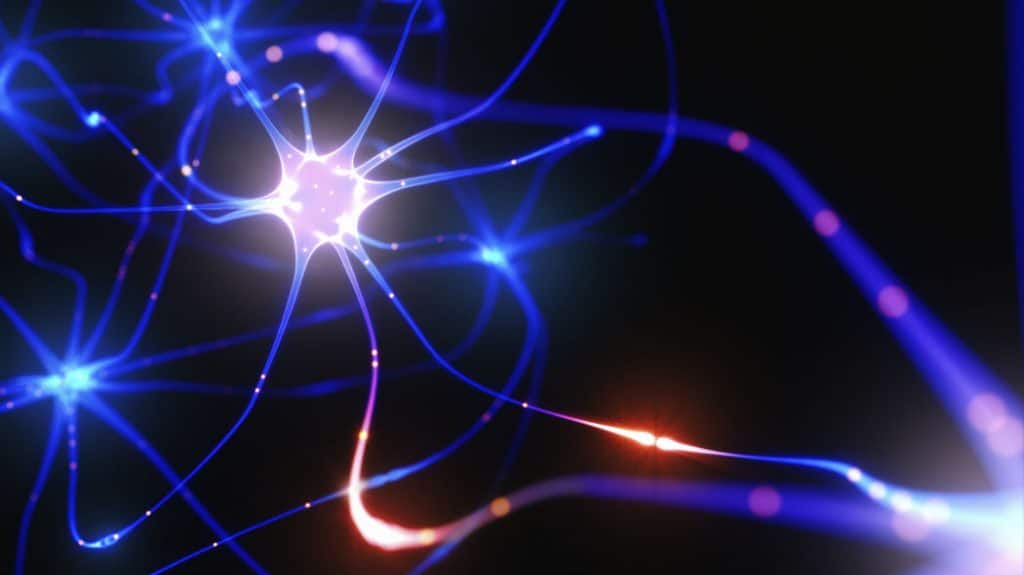
“Our job as computational neuroscientists is to use the language of mathematics to interpret experimental observations and build coherent models of the brain,” says senior co-author Dr. Pietro Verzelli, a postdoctoral researcher in Prof. Tchumatchenko’s group.
Here, the researchers developed a biophysical model of learning based on a biochemical feedback loop between astrocytes and neurons, recently discovered by Dr. Kirsten Bohmbach, Prof. Christian Henneberger and other researchers from the DZNE and UKB.
The biophysical model explains the learning deficits observed in mice with impaired astrocyte regulation and highlights the crucial role that astrocytes play in rapidly adapting to new information. By regulating levels of the neurotransmitter D-serine, astrocytes may facilitate the brain’s ability to adapt efficiently and reprogram its synaptic connections.
“Our mathematical framework not only explains experimental observations, but also provides new testable predictions about the learning process,” says first author Lorenzo Squadrani, a doctoral student in Tchumatchenko’s group.
This research bridges the gap between theoretical models of plasticity and experimental findings on neuron-glial cell interactions. It highlights astrocytic regulation as a physiological basis for dynamic synaptic adaptations, a central concept of synaptic plasticity.
“Our findings contribute to a better understanding of the molecular and cellular mechanisms underlying learning and memory and offer new opportunities for therapeutic interventions targeting astrocytes to improve cognitive functions,” says Prof. Tchumatchenko.
Astrocyte Networks in Mouse Brain Control Spatial Learning and Memory
Astrocytes form large networks of interconnected cells in the central nervous system. When these cell-cell couplings are disrupted in the brains of adult mice, the animals are no longer able to store spatial information. The astrocyte network is therefore essential for spatial learning and memory formation, as neuroscientists at the University of Zurich now demonstrate.
In the brain, neurons and astrocytes work together to process information and enable complex behaviors and cognition. Astrocytes have many functions such as controlling the blood-brain barrier, providing nutrients to nervous tissue, and supporting its repair. An interesting feature of astrocytes is that they form large networks of connected cells.
These couplings are made of specific membrane pores that are formed by a group of proteins called connexins. And through these connections, astrocytes can communicate with each other by exchanging various ions and small molecules.
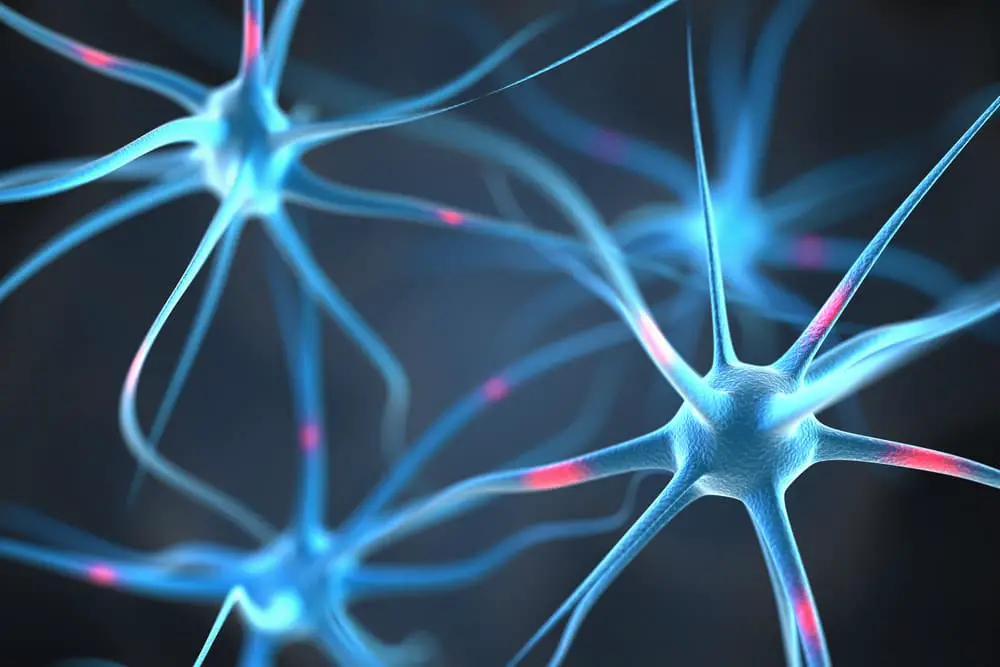
A team of neuroscientists led by Aiman Saab and Bruno Weber at the Institute of Pharmacology and Toxicology at the University of Zurich (UZH) has revealed that in the adult brain of mice, astrocyte coupling contributes to neural functioning in the hippocampus, a brain region involved in the formation of spatial memory.
“We found that in adulthood, an intact astrocyte network is essential for neural homeostasis, synaptic plasticity, and spatial cognition in this brain region,” says Aiman Saab, senior author of the study.
To clarify the functional relevance of the astrocyte network, the researchers generated a mouse model in which two key connexins responsible for connecting astrocytes could be selectively inactivated. Once the corresponding genes were inactivated, astrocytes lost their ability to maintain intercellular networks, and astrocyte-astrocyte coupling was disrupted within a few weeks.
Disruption of the astrocyte network altered the excitability of neurons in the hippocampus and their signal transmission to synapses. In addition, the strengthening of these specialized neuronal connections needed to store synaptic information was also impaired.
This was accompanied by significant deficits in the animals’ spatial learning and memory. “It is known that astrocyte functions are involved in the formation of cognitive abilities. Our study now shows that an intact astrocyte network is crucial for the formation of spatial memory in adult mice,” says Ladina Hösli, first author of the study.
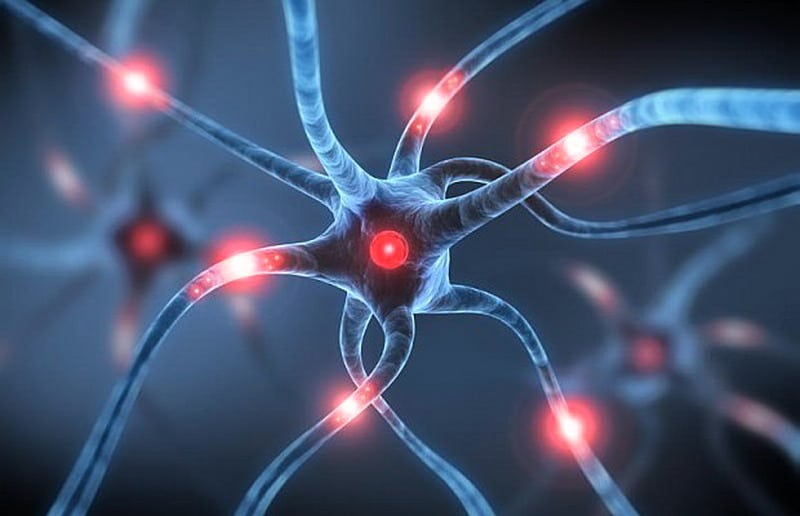
In addition, primary immune cells in the brain are also affected by the loss of astrocyte coupling. The activation of these so-called microglia observed in mice is similar to changes documented in neurodegenerative diseases such as Alzheimer’s and neuropsychiatric disorders such as depression.
“Not only did the astrocytes and microglia change their morphology, but we also found alterations in specific markers that are characteristic of disease-associated microglia,” says Hösli.
Because normal brain aging is also associated with changes in astrocytic coupling, these glial changes could contribute to age-related declines in learning and memory.
“Our study shows that in the adult brain, functioning astrocytic connexins and an intact glial network may be important for how astrocytes and microglia work together to maintain neural homeostasis,” says Aiman Saab.
In a next step, the researchers aim to understand how microglia functions are altered when astrocyte coupling is perturbed.
Astrocytes Help Orchestrate Synaptic Activity in Learning and Memory
Neuroscientists at RIKEN have discovered a surprising mechanism by which neuronal activity in mice is dynamically regulated, with signaling in some synapses increasing while other synapses are silenced, in a way that promotes the process of learning and memory formation. This finding provides new insights into the role that brain cells called astrocytes play in creating memories.
A team led by Yukiko Goda of the RIKEN Center for Brain Science sought to understand the neural processes underlying learning and memory formation. “One of our main goals is to understand how the strengths of individual synapses are set and changed dynamically,” says Goda.
In a 2016 study, Goda’s team used cell cultures derived from rat brains to study the behavior of simple systems in which multiple input neurons formed synaptic connections with the dendrite of a single receiving neuron. They determined that astrocytes (Fig. 1), a highly abundant population of cells that perform a variety of essential support functions in the brain, facilitated the strengthening of active synapses while weakening less active synaptic connections.
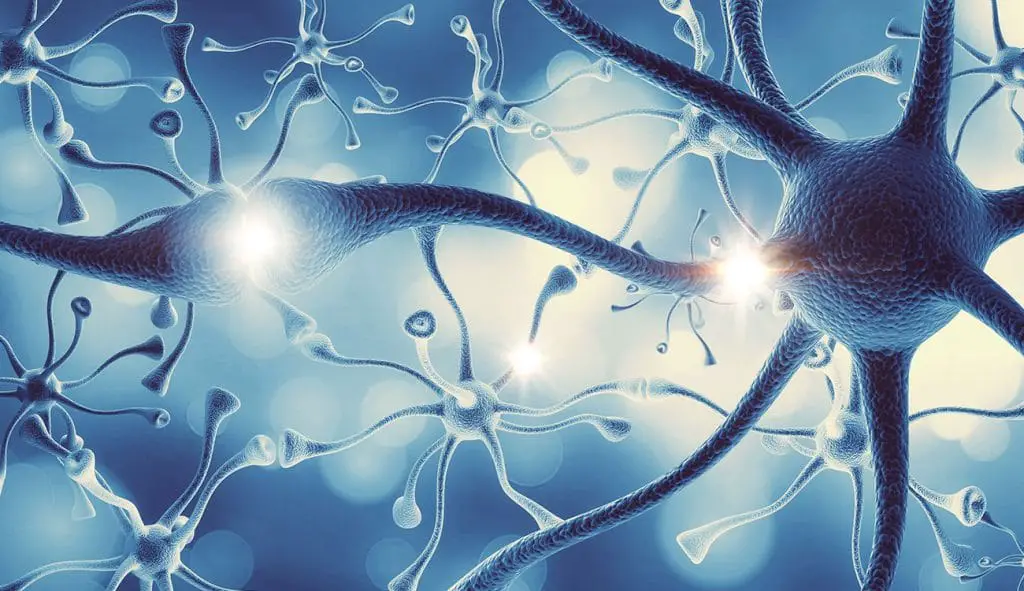
Now, the team has explored this regulatory mechanism in more depth. In particular, they focused on the role of receptors for the neurotransmitter N-methyl-D-aspartate (NMDA) in the mouse hippocampus, the region of the brain where memories are formed.
“NMDA is a well-established component of neuronal signaling in the hippocampus,” Goda explains. “But the idea of astrocytic NMDA receptors has been met with some skepticism.” However, his team’s previous work has provided compelling evidence that these receptors are directly involved in fine-tuning connections between neighboring neurons.
Goda and colleagues used various interventions to selectively interfere with NMDA receptor activity in mouse astrocytes. These treatments clearly affected activity on the presynaptic side of synapses, modulating the terminals of input neurons, rather than the dendrites of neurons that received those signals. As a result, synaptic activity between input and receiving neurons became more uniform overall, rather than dynamically shifting to favor activity at some synapses over others.
Mathematical modeling, done in collaboration with Tomoki Fukai’s team at the Okinawa Institute of Science and Technology Graduate University (OIST), revealed that these changes in synaptic function significantly reduced neural plasticity in the hippocampus, that is, the selective reinforcement of memories through the strengthening and weakening of synapses between neurons.
“Our work demonstrates that astrocyte signaling helps ensure a broad distribution of presynaptic forces,” says Goda.
The team is now trying to better understand the organization, activity, and distribution of NMDA receptors in hippocampal astrocytes and the broader influence of these non-neuronal receptors on animal behavior. “We want to find out whether mice with impaired astrocytic NMDA receptors show altered hippocampal network activity, and if so, whether these changes are related to spatial and contextual learning,” Goda concludes.
#Astrocytes #enhance #flexible #learning