Once upon a time the shape of the Solar system was a bit more doughy. Before it settled into a flattened disk, the distribution of dust and rocks was similar to a donut. This is the conclusion that scientists have come to after studying iron meteorites from the outer Solar System, finding that they can only be explained if its shape was once toroidal.
The research was published in the journal Proceedings of the National Academy of Sciences.
The shape of the Solar System
This is information that can help us interpret other emerging planetary systems and determine the order in which they assemble.
The formation of a planetary system around a star begins in a molecular cloud of gas and dust that drifts through space.
If a portion of the cloud becomes dense enough, it will collapse under its own gravity, rotating as it goes, becoming the seed of a growing baby star. As it rotates, material in the surrounding cloud is pulled into a circular disk that feeds the protostar.
Within that disk, smaller clumps form, which become protoplanetary seeds that continue to grow until they become full-blown planets or, which seems much more common, they stop growing, remaining smaller objects like an asteroid.
We’ve seen these disks over and over again around other stars, with the spaces carved out by planets swallowing up dust as they move.
But iron meteorites found in our Solar System tell another part of the story.
According to a team led by planetary scientist Bidong Zhang of the University of California, Los Angeles, the composition of asteroids in the outer Solar System requires that the cloud of material be shaped like a donut, rather than a series of concentric rings in a flat disk. This suggests that the early stages of the system’s coalescence are toroidal.
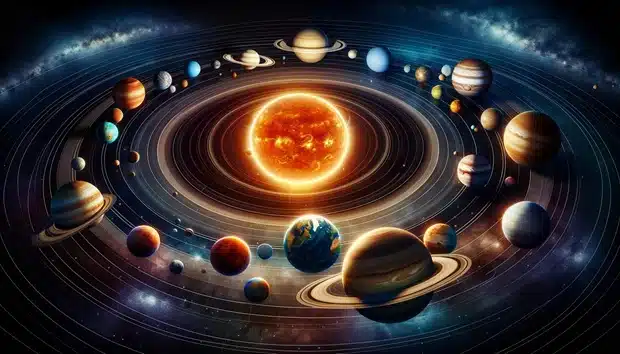
The iron meteorites in question, chunks of rock that have traveled a long way to Earth from the outer Solar System, are richer in refractory metals than those found in the inner Solar System. These are metals such as platinum and iridium, which can only form in a very hot environment, such as near a forming star.
This poses a bit of a problem, because these meteorites did not come from the inner solar system, but from the outer solar system, which means they must have formed close to the Sun and moved outward as the protoplanetary disk expanded. However, according to modeling by Zhang and his colleagues, these iron objects would not have been able to pass through the cracks in a protoplanetary disk.
According to their calculations, migration could have occurred more easily if the protoplanetary structure had been toroidal in shape. This would have pushed the metal-rich objects toward the outer fringes of the forming Solar System.
Then, as the disk cooled and planets began to form, the inability of rocks to pass through cracks in the disk would have acted as a very effective barrier, preventing them from falling back toward the Sun under the action of gravity.
“Once Jupiter formed, it is very likely that a physical gap opened up that trapped the iridium and platinum metals in the outer disk, preventing them from falling into the Sun,” Zhang says.
“These metals were later incorporated into asteroids that formed in the outer disk. This explains why meteorites formed in the outer disk (carbonaceous chondrites and carbonaceous iron meteorites) have much higher iridium and platinum contents than their inner disk counterparts.”
How did the solar system form?
4.5 billion years after our sun began its life, with a series of planets and smaller objects orbiting it. How did all the planets form and why did they end up in the orbits they did?
The formation of the solar system is a challenging puzzle for modern astronomy and a fantastic tale of extreme forces acting on immense time scales.
In the beginning, there was nothing. But it was nothing at all. All stars form from the collapse of nebulae, which are scattered clouds of gas and dust, and our sun (and solar system) are no different. Astronomers call it the “pre-solar nebula,” and of course it’s gone today, but we’ve seen enough solar systems form across the galaxy to get the general picture.
A nebula alone will not collapse into a solar system without something to set it in motion. In our case, we can thank a nearby supernova explosion, whose shock wave ripped apart the pre-solar nebula, causing it to begin contracting.
We can say that such a supernova exploded nearby, because supernovae release large amounts of certain radioactive elements, elements that are not normally found inside nebulae, but which we can see in our solar system today.
Once underway, the transition from nebula to solar system was irreversible. Over millions of years, the nebula contracted and cooled, eventually reaching the point where a proto-sun was surrounded by a thin, rapidly rotating disk of gas and dust.
Four and a half billion years ago, our sun was not quite the bright star it is today. It was compact and very, very hot, but it had not yet reached the critical densities and temperatures needed to sustain nuclear fusion in its core.
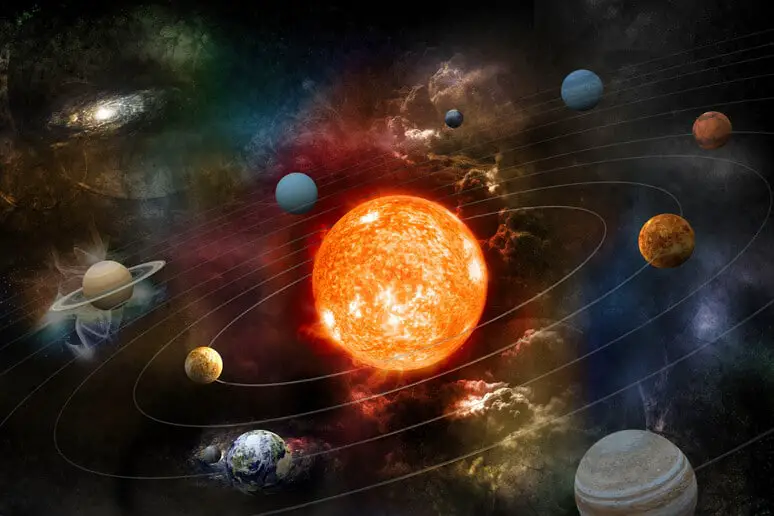
But while they were still in this embryonic stage, the planets began their slow and progressive formation.
Near the young sun, the heat and light were too intense for anything but rock to remain; ices evaporated, and dissolved gases such as hydrogen and helium simply flew away. What rock bits remained slowly melted, sticking together to form larger and larger clumps.
Eventually, given enough time (and the universe always has plenty of time to spare), those pieces formed planetesimals, tiny quasi-planets. There were a lot of them, and it was a pretty violent time for our solar system, as these planetesimals collided, shattered, and reformed countless times. Our Earth was hit by something nearly the size of Mars, and the debris from that impact eventually became our Moon.
Beyond what would later become the asteroid belt, however, planet formation took a different approach. Out there, it was cold enough for ices to survive, allowing planetary cores to grow to immense proportions in a short amount of time.
Those large cores were then able to suck in any surrounding material, such as hydrogen and helium, enveloping those worlds in thick, enveloping atmospheres. This is how giant planets were born.
Once the planets matured, however, all was not calm in the solar system. The inner rocky worlds had stabilized, and the sun had ignited nuclear fusion. But the outer giant planets were surrounded by swarms of parasites, the debris left over from the chaotic planet-building process.
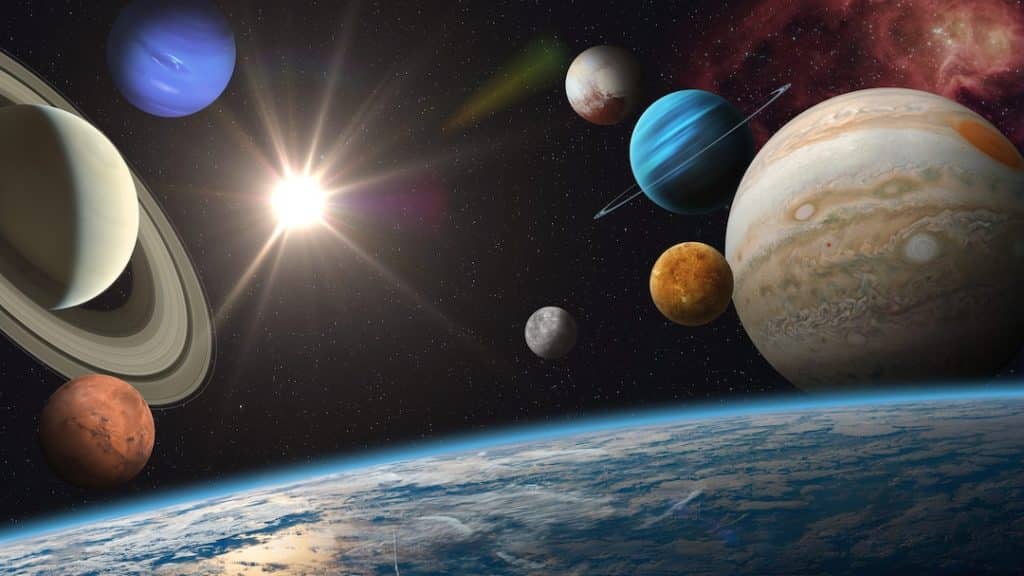
Astronomers suspect that our solar system’s four giant planets—Jupiter, Saturn, Uranus, and Neptune—first formed much closer together than they are today, and subtle interactions with the remaining debris surrounding them caused their orbits to shift. It took hundreds of millions of years for our solar system to be resculpted, and we’re not exactly sure how it all happened.
In one scenario, Jupiter and Saturn migrated inward toward the sun, which caused Uranus and Neptune to drift outward. In another scenario, the worlds in our outer solar system played a game of gravitational hot potato with a bonus fifth giant planet that was eventually kicked out altogether.
In yet another, Jupiter wanders nearly to the orbit of Mars before popping out, disrupting the otherwise placid orbits of the remaining outer worlds.
In any case, this latest shuffle has caused havoc. Astronomers think the migrating outer planets gave rise to an epoch called the Late Heavy Bombardment, a period of intense comet and asteroid impacts in the inner solar system about 4 billion years ago.
The shifting of the giant worlds disturbed all the remaining material in the solar system, sending it either safely to the icy outskirts or hurtling inward to cause problems for the rocky planets.
Despite the violence, it wasn’t all doom and gloom: the procession of comets raining down on the inner solar system brought an abundance of water to the rocky worlds, potentially making life possible—including our own—once the solar system stabilized, of course.
#shape #solar #system #changed #radically