The neurotransmitter dopamine it has often been linked to pleasure-seeking behavior and making stimuli paired with rewards (e.g., food, drinks) valuable. However, the processes through which this fundamental chemical messenger contributes to learning have not yet been fully understood.
The functions of dopamine
Researchers from the University of California at Los Angeles, the University of Sydney and the State University of New Jersey recently conducted a study aimed at better understanding how dopaminergic neurons (i.e. the brain cells that support neurotransmitter production) support reward-based learning.
Their results, published on Nature Neuroscience suggest that instead of representing the value attributed to different stimuli, these neurons contribute to the formation of new mental associations between stimuli and reward (or other neutral stimuli), which help us form cognitive maps of our environment.
“Our recent research has shown that the activation of neurotransmitter neurons acts as a teaching signal of the brain,” Melissa Sharpe, co-author of the paper, told Medical Xpress. “This occurs whenever something new or salient happens, which helps us learn to associate events together to create a new memory. Basically, we showed that dopamine neurons do this without making things ‘valuable’ or ‘good’ in and of themselves.”
This work contrasts with previous studies that had defined the neurotransmitter as the neurotransmitter that produces “happiness” or “pleasure.” However, if dopamine neurons do not carry value signals, they should not be able to attribute positive or pleasant qualities to specific experiences or actions.
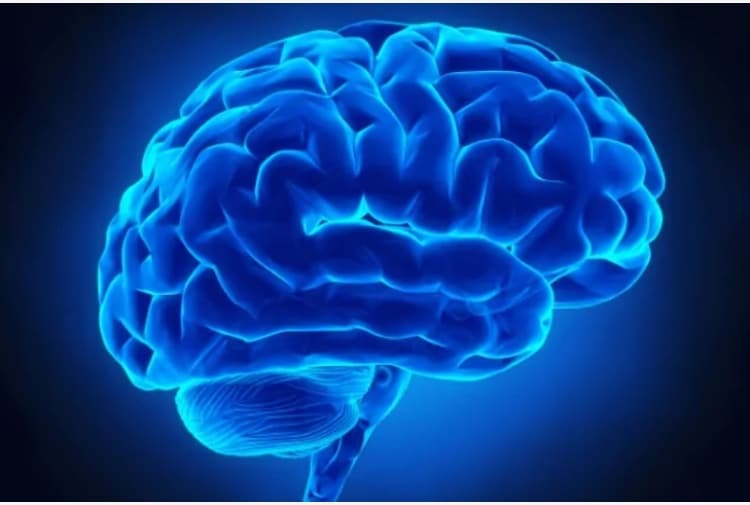
“We were wondering, if neurotransmitter neurons don’t carry a value signal, then how do they support intracranial self-stimulation, which suggests that dopamine neurons carry a value signal?” Dr. Sharpe explained. “Our experiments therefore aimed to answer the question: If neurotransmitter neurons actually have value in the context of intracranial self-stimulation, what is the cognitive representation that allows [loro] to do it?”
To answer this research question, Dr. Sharpe and her colleagues performed a series of experiments on rats. During these experiments, they used a Pavlovian-to-instrumental transfer procedure, a well-known experimental test designed to elucidate the cognitive representations that drive animal or human behavior.
“We teach rats that a signal (for example, a tone or a click) leads to a particular outcome (for example, neurotransmitter stimulation or a food pellet),” Dr. Sharpe said. “So when the tone or click is played, one of these outcomes occurs (e.g. tone -> neurotransmitter stimulation). Then we teach them that they can achieve these results by pressing one of two levers.
If the tone makes them think of the ‘specific’ outcome it has been paired with (e.g., neurotransmitter stimulation), they will selectively increase the lever press associated with neurotransmitter (and not food) stimulation.”
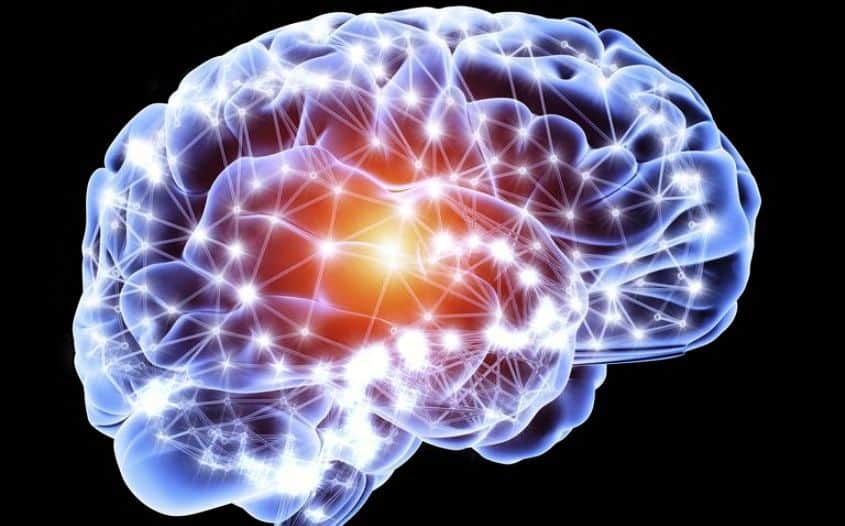
Experiments conducted by Dr. Sharpe and her colleagues produced several interesting results.
First, the researchers found that a physiological firing rate of neurotransmitter neurons did not support intracranial self-stimulation in a way that would suggest that neurotransmitter neurons carry a valuable signal.
The researchers observed that if they activated dopaminergic neurons above this physiological rate, the activation of these neurons could function as a specific sensory target towards which the animals would exhibit behavior.
That is, the high firing rate of dopamine neurons could function as a reward that ultimately drove the rats to engage in pleasure-seeking behaviors associated with the so-called Pavlovian-instrumental transfer effect.
“This suggests that when dopamine neurons fire in everyday life, they don’t value things,” Dr. Sharpe explained. “Instead, they work to help us form new memories or how things in our environment are related.
In cases where dopamine neurons fire more than they should (for example, when taking drugs of abuse), this can be encoded in the brain as a rewarding event that makes us more likely to seek drugs in the future.”
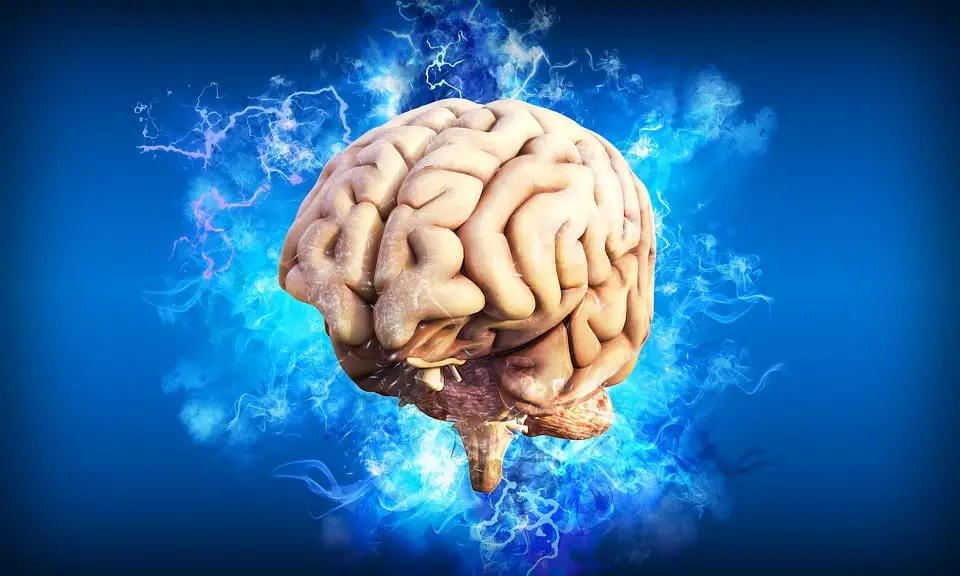
Overall, this recent study by Dr. Sharpe and her colleagues could contribute greatly to the understanding of dopamine and its role in reward-based (i.e., reinforcement) learning. In particular, their findings suggest that dopamine neurons do not carry value signals that attribute pleasure or happiness to environmental stimuli.
In the future, they could pave the way for additional experiments aimed at further validating the team’s findings or examining the unique contribution of specific neural circuits that produce dopamine.
“Our team is now interested in how different dopamine circuits contribute to different types of learning and how this helps us create a complex but unified representation of our environment,” added Dr Sharpe.
What happens to the dopamine system when we experience adverse events?
A study at the Netherlands Institute for Neuroscience examined how the dopamine system processes aversive unpleasant events.
The dopamine system is known to play a crucial role in motivation, learning and movement. One of the main functions of dopamine is to predict the occurrence of rewarding experiences and the availability of rewards in our environment. In this context, the dopamine system informs our brain about so-called “reward prediction errors”, i.e. the difference between the reward received and the expected one.
Dopamine neurons become more active when a reward arrives unexpectedly or if it is larger than expected, and show depressed activity when we receive less reward than expected. These error signals help us learn from our mistakes and teach us how to achieve rewarding experiences.
While a large number of studies have focused on the relationship between dopamine release and rewarding stimuli, few have examined the effect of unpleasant and aversive stimuli on dopamine. Although the results of these few experiments have been inconsistent, it has become clear that aversive stimuli impact the dopamine system.
But there is an active debate among neuroscientists about what precise role dopamine neurons play in processing aversive stimuli: Does their activity change in response to aversive events? Do they predict adverse events? Do they encode an aversive prediction error?
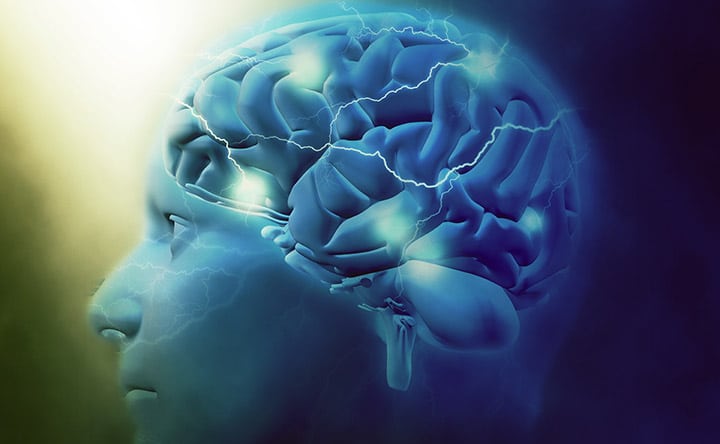
The team around Ph.D. student Jessica Goedhoop and group leader Ingo Willuhn exposed rats to white noise in combination with stimuli involving white noise, while measuring the release of dopamine in the brain. White noise is a well-known example of an unpleasant auditory stimulus for rats.
The researchers found that dopamine release gradually decreased during exposure to white noise. Furthermore, after a coherent presentation, stimuli that occurred a few seconds before exposure to white noise began to have the same depressant effect on dopamine neurons. However, unlike how it processes rewards, dopamine did not encode a prediction error for this aversive stimulus.
Overall, this new study demonstrates that the dopamine system helps the brain anticipate the occurrence and duration of unpleasant events, but without accounting for prediction errors.
Group leader Ingo Willuhn said: “This is a very thorough and systematic study that takes many variables into account. The findings give us a better understanding of the role of dopamine release in the processing of aversive events. There is growing interest in the role of dopamine in aversion. We used a new aversive stimulus that allowed us to conduct a more in-depth analysis of dopamine than previously possible.”
Addictive drugs hijack and amplify dopamine signals and induce exaggerated and uncontrolled effects of dopamine on neuronal plasticity. This study brings us closer to understanding the mechanism underlying this pathological phenomenon.
#Dopamine #contributes #learning